This is a summary and explanation of the following research paper:
Microplastics in the Food Chain
- Published In: Life
- On: Dec 6, 2021
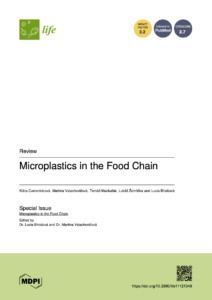
Key Takeaways:
- Microplastic contamination occurs at various levels of the food chain, from lower trophic levels (like zooplankton and copepods) to higher trophic levels (such as fish, seabirds, and mammals). This contamination can occur either through direct consumption or trophic transfer.
- The paper explores how microplastics enter the human food chain primarily from contaminated foods and can potentially impact human health. While a large fraction of consumed micro- and nanoplastics is excreted, smaller microplastics can penetrate deep into the organs, posing greater risks. The long-term health effects of microplastics are still relatively unknown but may include oxidative stress, cytotoxic effects, and disruption of energy balance and immune system functions.
Research Paper Summary
The research paper “Microplastics in the Food Chain” focuses on several key points regarding the impact of microplastics on the environment and human health:
Widespread Contamination of Microplastics
The paper highlights that microplastics are a widespread contaminant found in almost every part of the environment. Since the 1950s, the plastic industry has generated waste that now counts in the millions. A significant portion of plastic consumption is used for packaging materials, including those in the food industry. The contamination of the environment with microplastics and nanoplastics is emerging as a global problem, posing risks to animal life, the food chain, and public health.
Microplastics in the Human Food Chain
The main goal of the review is to summarize the occurrence of microplastics in the human food chain. It emphasizes the role of microplastics as vectors for various organic micropollutants and microorganisms and mentions the health consequences associated with the consumption of microplastics.
Toxic Additives and Microorganisms on Microplastics
The paper discusses how microplastics can serve as carriers for the spread of toxic chemicals in the marine environment. Additionally, it talks about the association of microorganisms with microplastics, highlighting the potential for plastics to act as vectors for pathogenic microorganisms that can enter the digestive tract of fish and other marine life.
Microplastic Contamination Across the Food Chain
It is reported that microplastic contamination occurs at various levels of the food chain, from lower trophic levels (like zooplankton and copepods) to higher trophic levels (such as fish, seabirds, and mammals). This contamination can occur either through direct consumption or trophic transfer.
Effects on Human Health
The paper explores how microplastics enter the human food chain primarily from contaminated foods and can potentially impact human health. While a large fraction of consumed micro- and nanoplastics is excreted, smaller microplastics can penetrate deep into the organs, posing greater risks. The long-term health effects of microplastics are still relatively unknown but may include oxidative stress, cytotoxic effects, and disruption of energy balance and immune system functions.
Conclusions and Recommendations
The paper concludes that while data on the occurrence of microplastics in the food chain are growing, they are still insufficient, and there is a lack of a uniform methodology for determining microplastic contamination in food. It recommends introducing legislation to regulate the use of primary microplastics and their release into the environment. Additionally, it suggests that more intensive research is needed on the impact of microplastics on the food chain and human health, as well as the development of new technologies for microplastic degradation and raising public awareness.